Laboratory for Spectroscopy is equipped with two triple Raman spectrometers (Jobin Yvon T64000 and TriVista 557) and Brillouin spectrometer (two Tandem Fabry-Perot Interferometers TF-1, JRS Scientific), along with three lasers emitting different excitation wavelengths, cryostats, and a cooling-heating stage. Additionally, this laboratory is equipped with two piezo-strain cells. One of these cells is custom-made in our facility, while the other is commercially available from Razorbill Instruments. Strain cells can be installed in both of our cryostats and coupled with Raman experiments but also with the other techniques available in the Center for Solid State Physics and New Materials.
Raman Spectrometers
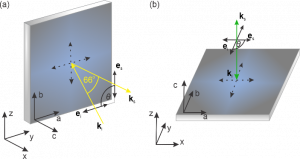
Figure 1: Schematic representation of (a) pseudo-Brewster configuration and (b) backscattering configuration.
Two Raman spectrometers are available in our laboratory, both upgraded to enhance the analysis of various sample types, ranging from those exhibiting strong electronic correlations (such as quasi-low dimensional materials and superconductors) to thin films, nanostructured
samples, and even biological specimens (tissues, cells etc.). Both spectrometers enable transitions between additive and subtractive modes. In additive mode, high spectral resolution and linear dispersion are delivered, whereas in the subtractive mode, high stray light rejection is ensured. In addition, two scattering geometries are available: micro-Raman backscattering and macro-Raman configuration, where the incident light strikes the sample at a pseudo-Brewster angle which is more convenient for detection of low-energy excitations.
TriVista 557
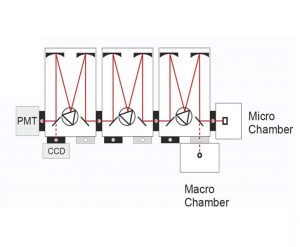
Figure 2: Schematic representation of Tri Vista 557 in micro- and macro-Raman setup in triple additive and subtractive mode ( https://www.s-and-i.de).
The TriVista 557 (Figure 2.), a triple spectrometer designed for Raman and photoluminescence measurements across UV, VIS, and NIR spectral ranges, owns a high resolution and stray light rejection capabilities. Its unique optical configuration enables reconfiguration for double or even single spectrometer operations.
Operating from 185 nm to 2.2 μm, the TriVista 557 achieves exceptional spectral resolution, reaching as low as 4 pm in the VIS range (500 nm). The first and second stage of TriVista feature 500 nm focal length, while its length in the third stage is 750 nm. Its high stray light rejection allows Raman spectra to be captured close as 5 wave numbers of the Rayleigh line. Nine gratings (in three stages), ranging from 300 to 2400 grooves/mm, enable the collecting of Raman spectra in different spectral ranges and resolutions.
The physical mechanism behind additive-subtractive mode switching is the direction of the grating’s rotation. In additive mode, both first and second stage gratings rotate clockwise, enhancing dispersion; in subtractive mode, the first stage grating rotates clockwise while the second stage grating rotates counter-clockwise, effectively canceling out dispersion from the first stage.
Jobin Yvon T64000
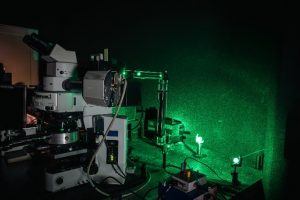
Figure 3: Optical path and microscope as a part of Jobin Yvon T64000.
The Jobin Yvon T64000 (Figure 3.) system consists of a double pre-monochromator (1st and 2nd stage) and a spectrograph stage (3rd stage) with a focal length of 640 nm. The pre-monochromator operates as a twin monochromator in a subtractive mode, acting as a tunable filter within the spectral range defined by the scanning mechanism and the gratings. The spectrograph stage serves as a disperser. Equipped with three holographic 1800 grooves/mm gratings, the T64000 system defines a mechanical range of 0-950 nm. Transitioning between the additive and subtractive modes is achieved by inverting the beam using a system of mirrors.
Brillouin Spectrometer
Brillouin scattering is an inelastic light scattering technique, similar to Raman spectroscopy. However, in Brillouin spectroscopy, the energies of the probed excitations are significantly lower than those in Raman spectroscopy, typically lying well below 50 cm⁻¹. The range of excitations that can be explored with Brillouin spectroscopy includes acoustic phonons, magnons, spin waves, surface ripples, and diffusive excitations, among others.
As the energy of the scattered radiation closely aligns with the initial laser line energy, often differing by less than an inverse centimeter, a grating monochromator cannot be used as a spectrograph. Instead, a Fabry-Perot interferometer (either plane or spherical) is used. The plane Fabry-Perot interferometer comprises two parallel round mirrors (typically made of glass or quartz) positioned at a fixed distance as (L). These mirrors are coated with a material that predominantly reflects incident light while allowing only a small fraction to transmit. Through the interference of light after multiple reflections, wavelength maxima occur at λ=2L/p. By predominantly transmitting light of these wavelengths, individual wavelengths are effectively separated from the rest of the scattered radiation.
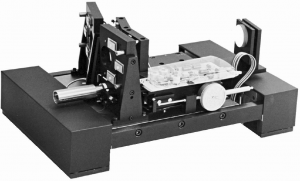
Figure 4 : Tandem Fabry Perot Interferometer TF-1. (http://www.jrs-si.ch)
Our Brillouin scattering system, the Tandem Fabry-Perot Interferometer TF-1 (JRS Scientific) (Figure 4.), consists of two interferometers positioned with their main axes nearly parallel, exhibiting a slight offset angle φ to ensure their mirror distances are nearly identical. The light undergoes three passes through each interferometer (six passes in total) significantly enhancing the contrast. Both interferometers are piezoelectrically scanned and their operations are synchronized by mounting their scanning mirrors on a shared scanning stage. Additionally, positioning the interferometers in close proximity renders the system less sensitive to temperature fluctuations. The mirror spacing can be adjusted within the range of 0-50 mm, and small mirror movements with scanning amplitudes of up to 2 μm are achievable. Both interferometers are isolated from the low-frequency vibrations.
Excitation Sources

Figure 5 : Laser lines (in nm) emitted by Coherent Innova 70C
Laboratory for spectroscopy is equipped with three lasers of different kind and wavelengths of emitted light.
- Coherent Innova 70C Spectrum - mixed Ar+/Kr+ gas laser, emitting six different laser lines (from blue to red, Figure 5.)
- Coherent Verdi-G solid state laser emitting 532 nm laser line
- Coherent Compass 315M diode-pumped Nd:YAG laser emitting 532 nm laser line
Cooling and heating systems
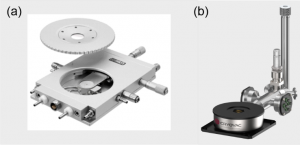
Figure 6: (a) Linkam THMS600 (http://www.linkam.co.uk). (b) Cryovac Konti He-flow cryostat (https://www.cryovac.de).
Linkam THMS600
The THMS600 is heating/cooling stage (Fig. 6. (a)) used in many applications where high heating/cooling rates, less than 0.1°C accuracy and high stability. It is equipped with a CI94 temperature controller and a LNP94 Liquid Nitrogen Pump. It operates in wide temperature range from -196° to 600°C. Recycled dry nitrogen gas is used to purge the sample chamber and upper lid window surface of condensation. The precise control of liquid nitrogen flow allows cooling rates as fast as 130°C/min or as slow as 0.1°C/min.
Cryovac Konti cryostat
Cryovac Konti He-flow cryostat for micro-spectroscopy (Fig. 6. (b)) enables low temperature measurements from 4K up to room temperature. It is supplied with liquid helium or nitrogen via vacuum isolated transfer line. This ultra-low in vibration and compact in size cryostat can be used in various configurations and orientations.
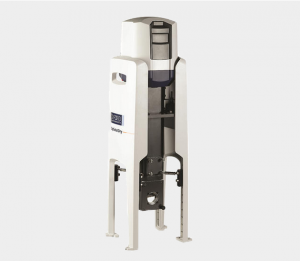
OptistatDRY BNL closed cycle cryostat (http://www.andor.oxinst.com)
OptistatDRY BLV cryostat
OptistatDRY BLV closed cycle cryostat works in a temperature range from 3K up to 300K with stability of ±0.1 mK. Has a large optical access of 28 mm diameter clear view f1 and flexible wiring options. Typical RMS displacement vibration of this cryostat is 10 microns at the sample position.
Piezo-strain cells
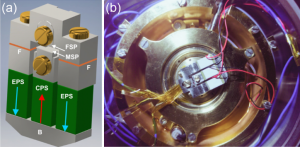
Figure 7: (a) A three-dimensional schematic presentation of designed piezo-strain sample holder with indicated components. (b) Piezo-strain cell coupled with Cryovac Konti cryostat.
Custom-made strain cell
Generally, piezoelectric elements are widely employed for that purpose in a broad temperature range. At the same time, they operate at cryogenic temperatures as well. The holder was designed according to the model presented in Ref. [C. W. Hicks et al. “Piezoelectric-based apparatus for strain tuning”, Rev. Sci. Instrum. 85, 065003 (2014)], with several modifications.
The three-dimensional picture of the designed sample holder is displayed in Fig. 7(a). The central part of the holder comprises three piezoelectric stacks, namely Piezo-pst1 from Piezomechanik GmbH, constructed from piezo-ceramics (PZT lead zirconium titanate) with a cross-section of 5×5 mm² and a length of 9 mm. These stacks can withstand a maximal load force of 2000 N, exhibit a stiffness of 120 N/µm, and operate within a temperature range from -273°C to approximately 120°C, with a voltage range from -30 V to 150 V.
The sample extension-compression mechanism operates as follows: when the compressive piezoelectric stack (CPS) located centrally is extended along the red arrow indicated in Fig. 1(a), the moveable sample plate (MSP) is pushed towards the fixed sample plate (FSP), resulting in sample compression. Conversely, when the two extension piezoelectric stacks (EPS) located externally are extended along the blue arrows shown in Fig. 1(a), the entire bridge (B) and the MSP move away from the FSM, leading to sample extension.
In order to prevent undesired bending and/or tension in the transverse plane, the body of the sample holder is made from titanium because of its low thermal contractions and the thermal contraction coefficient similar to piezoelectric stacks. Still titanium has lower thermal contraction coefficient. Therefore, in order to increase contraction of the holder at low temperatures, two thin copper foils (F) are inserted at the contact interface between two holder’s segments. Screws (four of them are depicted in Fig. 1) are made of brass which contracts even more than titanium at low temperatures which effectively leads to additional tightening of the whole platform. Samples are glued to the sample holder by a special epoxy which operates well at cryogenic temperatures and does not influence the strain determined by piezoelectric stacks.
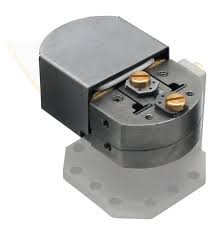
Figure 8: Razorbill Instruments CS100 strain cell
Razorbill Instruments CS100 strain cell
The CS100 strain cell is ideal for experiments on strongly correlated electron systems, thanks to its operational capabilities at cryogenic temperatures and under strong magnetic fields. It features a maximum applied displacement of ±3 µm and a maximum applied force of ±45 N, along with a feedback sensor for applied displacement.